How so-called ‘junk DNA’ affects cancer risk
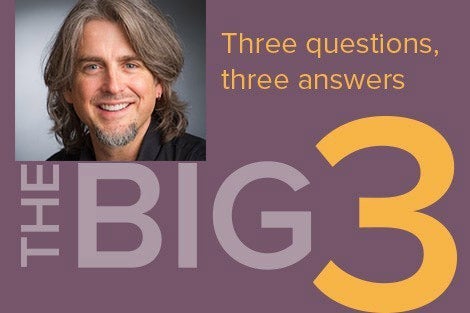
January 29, 2020 – John Quackenbush, Henry Pickering Walcott Professor of Computational Biology and Bioinformatics and chair of the Department of Biostatistics, is trying to understand how our genes influence cancer risk. In a recent research paper, he and his colleagues explored an often-overlooked segment of the human genome that may have a profound impact on cancer formation and prevention.
You recently published a study that examined how areas of the human genome that are sometimes referred to as “junk DNA” might influence cancer risk. What does “junk DNA” actually refer to?
The term junk DNA has been around for a long time, but I think in a lot of ways it’s a misnomer. It was a term that was applied to DNA whose function we don’t really understand. If you think about the human genome, it is 3 billion base pairs in length. That’s a huge number. But if we look at the amount of DNA that actually encodes for proteins, it’s somewhere on the order of 1-1.5% of the DNA in our cells. So more than 98% of DNA doesn’t have a clear function, and that’s what some people refer to as “junk DNA.” What our new research has shown is that a lot of this so-called junk DNA, or what we refer to as non-coding DNA, actually plays an important regulatory role. I think calling it junk DNA mischaracterizes it. One man’s junk is another man’s scientific treasure.
Can you unpack the idea that segments of non-coding DNA play a regulatory role when it comes to cancer?
As I mentioned, 1-1.5% of our DNA encodes the genes that we think make us who we are. But as we’re learning, the rest of our DNA actually plays a very important role in processes that turn those genes up and down, or as we say, it regulates how intensely those genes are expressed. This regulatory process is one of the things that makes us who we are, including helping to determine our individual risk for developing cancer.
When we think about genetic risk of cancer, we often think of mutations in genes like BRCA 1 or P53 or KRAS. But the truth is, a gene mutation alone isn’t enough to explain whether someone will develop cancer. There are millions of other variants—DNA differences between you and me—that fall into what people call junk DNA, and each of those variants can tug a cancer gene’s expression up or down. The reason I’m using the word tug is because any of these genetic variants by itself has a really, really, really tiny effect. But together, these millions of variants can have a significant effect. Not every variant affects every gene, but many variants can affect each individual gene.
This new research illuminates how groups of variants, or “networks,” in non-coding DNA can work collectively to influence cancer risk. It makes me think of the Lilliputians tying down Gulliver on the beach. They all have these tiny threads and each thread is only going to exert so much force. But thousands of them together can immobilize a giant. Or in the case of DNA variants, alter the biological systems that control our cells.
What’s the translational potential for this research? In the future, could better understanding of these regions of non-coding DNA lead to better cancer treatments or diagnostics?
One of the important things this research does is that it gives us a better framework to estimate individual cancer risk. At the moment, there are a small number of genetic variants that we can use to do a reasonable job of estimating disease risk, but they are not perfectly predictive. Far from it. The methods we’re developing start to give us ways to have a more robust understanding of an individual’s cancer risk.
The other thing this research does is provides a framework to better predict which individuals are likely to respond to different therapies. The members of my research group and I were recently discussing the next steps in our research, and we agree that a key area of emphasis will be using what we’ve learned to make more accurate and reliable predictions than methods that have been used to date. We are very excited about the potential and some early work indicates that understanding the collective effects of genetic variants is essential if we are going to be successful.